1.
All
continents that moved (North
America, South America, Antarctica, Australia, India,
Southeast Asia)
went
directly away from one unique central point.
It is just north of Madagascar in the Western
Somali Basin, centered on Wilkes Rise and the
Aldabra Islands.
2.
At
that central point is a feature unlike any other
on the ocean floor. It appears to be a giant crater
over 600 miles in diameter, based on the distance from
the clearly visible central uplift to the edge marked
by the Comoros and the Amirante Trench.
By comparison, Chicxulub crater is 112 miles in
diameter.
Click
pictures to enlarge
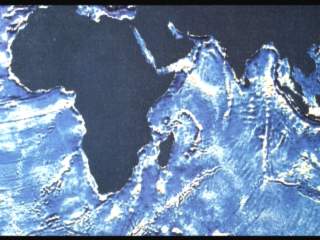
3.
The Earth flexes as the Moon revolves around it. The
place where it is the least flexible is shown in dark
blue below. Something has changed the layers deep
below the surface there, and geologists have no idea
why. But it is right over the giant crater.

This solid Earth (body) tide
projection was "generated from the actual history of the tidal
forcing". It is a measure of elasticity.
The image was computed using the 3-D model SPRD6 of
Ishii and Tromp. --Latychev, Konstantin, Jerry X. Mitrovica,
Miaki Ishii, Ngai-Ham Chan, James L. Davis. 2009. Body tides on
a 3-D elastic earth: Toward a tidal tomography. Earth and
Planetary Science Letters, Vol. 277, No. 1-2, pp. 86-90.
"The tidal response
of the Earth reveals a great deal about its inner structure."
"The calculations show that the perturbation in surface
deformation is large, with contributions from both elastic moduli
and density heterogeneity." --Ishii, Miaki, Konstantin
Latychev, Jerry X. Mitrovica, Ngai-Ham Chan, James L. Davis. Body
Tides on a Three-Dimensional Elastic Earth: Toward a Joint Tidal
and Seismological Tomography. American Geophysical Union, Fall Meeting
2008, abstract #DI11A-08.
4.
In their original positions next to the crater,
the coasts of Madagascar, Africa, and Australia are
rounded
(bights).
(The
brown segment shows the former position of the Horn
of Africa).
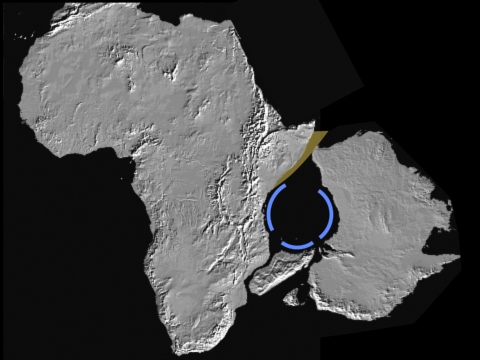
5.
Around the crater are features made by powerful
forces.
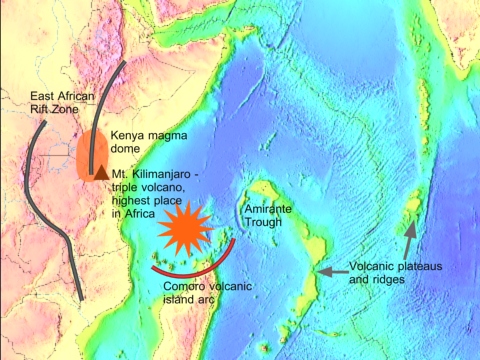
6.
Minor mountains on the continents that moved are
on the side nearest the crater.
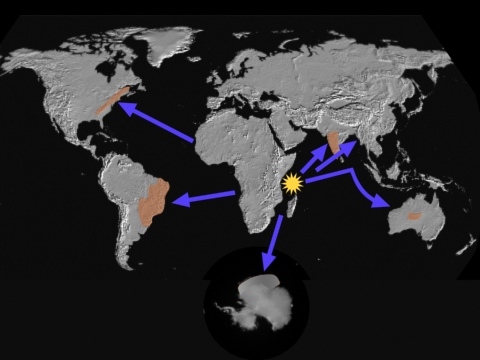
7.
Major mountains are on the side farthest from
the crater.
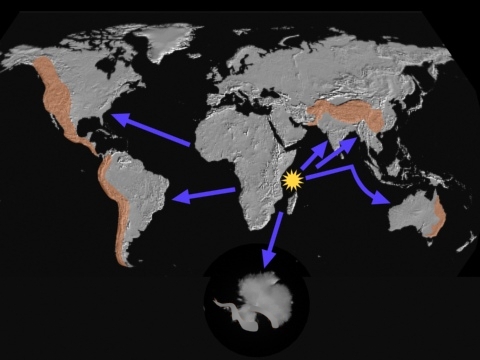
8.
If continental crust were being carried about
on brittle plates, even though it is flexible it would
keep its shape. But if continental crust slid
on top of a stationary surface, it would stretch
and bend, especially where it is narrow.
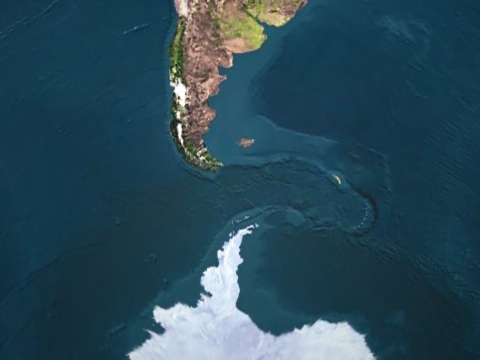
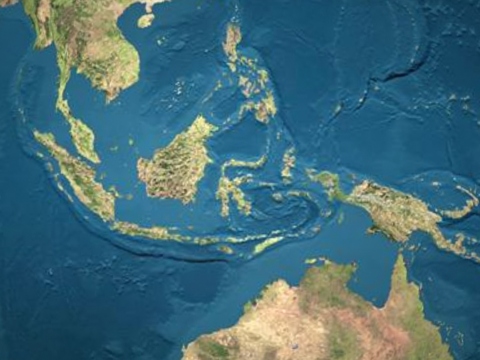
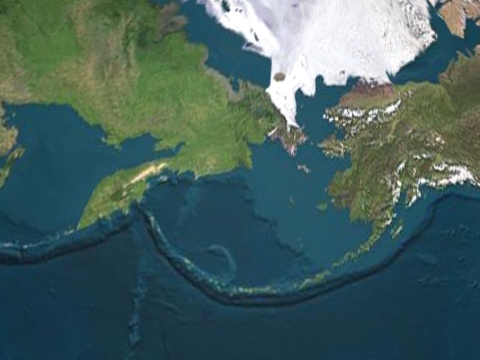
9.
The Shock
Dynamics event left us with Plate Tectonics
Slow Motion
If the work attributed
to Plate Tectonics over 200 million years was actually accomplished
by the Shock Dynamics event in 26 hours, what causes the few centimeters
per year of motion of the "plates" measured by GPS?
"The fact that
plate velocity on Earth does not depend on plate size and that the
velocities of the fast plates do not depend on length of the subduction
boundary suggests that it is the system, not the individual plate,
that dictates plate motions." "The possibility that
has been overlooked is that the plates define and organize themselves
by mutual interactions of the whole plate system and that minimization
of dissipation in the lithosphere may be the organizing rule."1
A number of researchers5,6,7,8
have picked up on the fact that the lithosphere constantly drifts slightly to the
west relative to the mantle below it. According to Harvard's
Jerry Mitrovica, magnetic coupling between the Earth's
iron core and its rocky mantle has been slowing the
rotation of the lithosphere.9
"In the hotspot
reference frame a 'westward' rotation of the lithosphere can be
observed. The origin of this net rotation of the lithosphere
is still under debate, but it should range between 4.9 cm/yr and
13.4 cm/yr at its equator."3
In the differential rotation model, the lithosphere and outer
core show a net westward drift, while the mantle and inner core
move eastwards.5,7
"Because the Antarctic
plate is centered on the Earth's south pole and is nearly surrounded
by ocean ridges, it can be regarded as an inertial reference with
regard to Earth's rotation axis."6
Where the crust is thickest, such as under mountain ranges,
there is the most contact with the eastward moving mantle.6
Proponents of differential
rotation find
that the axis of rotation of the lithosphere relative to the mantle
is different than the rotational axis of the whole Earth:
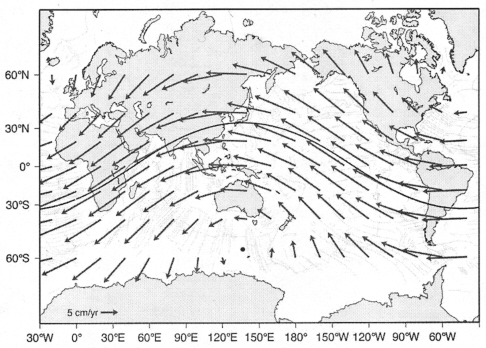 Global
lithospheric net rotation relative to the mantle assuming a
mid-asthenospheric source of the Pacific plumes.3
Transferring the rotational
path in the above illustration to a globe yields the red ring below.
For reference, the blue ring follows the equator. The
orange button at the top marks true north; the purple button marks
present-day magnetic north; and the red pegs mark the axis of the
red ring.
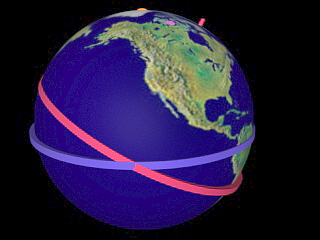
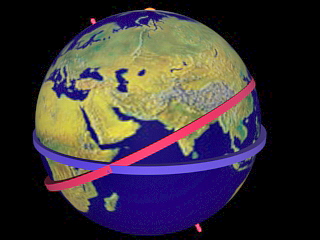
The rotational path
above (red band) "has an angle of about 30 degrees with respect
to the equator, close to the revolution plane of the Moon about
the Earth (28 degrees), although this overlap is intermittent."5
This is significant, because tidal force provides a constant
westward pull on the lithosphere.
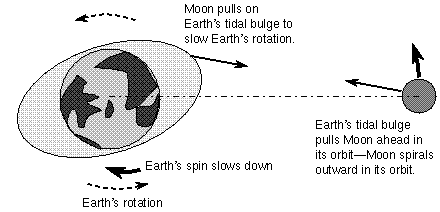
The approximately
"2 - 3 degree tidal lag angle between the tidal bulge and the
gravitational alignment between the Earth and Moon determines a
permanent torque toward the "west", opposite to the eastward
rotation of the planet. This torque is considered responsible
for the secular deceleration of the Earth, and acts directly on
the lithosphere." "To conserve the angular momentum
of the system, the Earth's deceleration is mostly compensated by
the enlarging of the Moon's orbit, at a rate of 38.2 + 0.7
mm/yr."5
"The solid Earth
tides have a well-known vertical oscillation of 300 - 400 mm/12
h 25', but they also have a relevant 150 - 200 mm/12 h 25' horizontal
swinging [i.e. stress loading]. Under a permanent torque,
this oscillation may induce a tiny strain in the upper asthenosphere,
say 0.1 -0.2 mm. The cumulative effect of this small horizontal
motion... may well reach several centimeters (7 - 14) per year".
"The advantage of this mechanism is to act contemporaneously
all over the lithosphere."5
"The idea of
tidal drag as the driving mechanism for plate tectonics is particularly
intriguing because it is energetically feasible. In fact,
the dissipation of energy by tidal friction is slightly larger (1.6
x 1019
J/yr) than the energy released by tectonic activity (1.3 x 1019
J/yr)."6
When differential
rotation was proposed as a driving mechanism in the early years
of Plate Tectonics theory, opponents "discarded Earth's rotation
as the cause of the westward drift, claiming that the viscosity
necessary to allow decoupling between lithosphere and mantle...
is too low when compared with present-day estimates of the asthenosphere
viscosity."
"Tidal torque provides a sufficiently energetic mechanism
to drive this motion, but requires a mechanical decoupling between
the lithosphere and the deeper mantle that is incompatible with
current understanding of upper-mantle viscosity."
However, "the mantle very likely has a nonlinear rheology,
and the viscosity of the asthenosphere can be far lower in thin,
undetected layers." "A thin, low-viscosity layer
(shear zone) could accommodate this motion."6
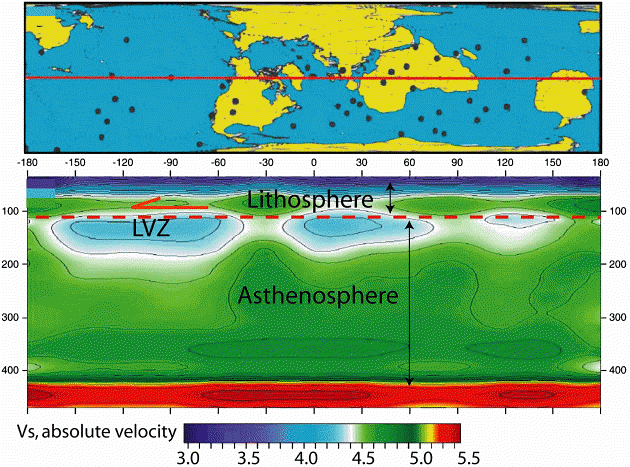
The lower image
is a cross-section of the Earth along the red line in the upper
image. The black dots in the upper image are stations
from which seismic readings were taken. The lower image is
called an "absolute shear wave velocity model" that displays
the seismic readings. It
shows "a pronounced global Low Velocity Zone (LVZ) at the top
of the asthenosphere, which is strongest underneath the oceans,
but is also clearly identifiable underneath the continents. This
level is here inferred as the main decoupling zone at the base of
the lithosphere, possibly having an internal sub-layer with ultra-low
viscosity, much lower than the average asthenosphere."5
"The viscosity
in... the LVZ of the asthenosphere can be much lower than present-day
estimates of asthenosphere viscosity based on post-glacial rebound,
because horizontal viscosity under shear can be several orders of
magnitude lower than the vertical viscosity computed by averaging
the whole asthenosphere."5
There is some evidence
in two studies of North America to suggest that there is an ultra-low
velocity zone at the top of the LVZ, due to shear stress lowering
the viscosity of the upper asthenosphere, using the standard assumption
of non-linear rheology of the mantle.5
In fact, tests on
olivine crystals by a French and German team of scientists2
found "that previously published high-temperature flow laws
(i.e., power flow law) overestimate the strength of lithospheric
mantle, since all samples deformed under stresses that were significantly
lower than those predicted by these flow laws." Their
research was aimed at understanding "the plasticity of the
uppermost part of Earth's mantle, just below the Mohorovicic discontinuity
(Moho), which separates the crust and the mantle". At
"pressures and temperatures relevant to the uppermost mantle
in continental and oceanic plates (pressures below 3 gigapascals
and temperatures in the range of 500 to 1000 degrees Centigrade)",
their findings indicate "a weak strength of Earth's uppermost
mantle lithosphere".2
In the Shock Dynamics
view, the "frozen" edges of crustal waves in the Pacific
and beneath the leading edges of continents that moved became what Plate Tectonics
calls plate boundaries. They are
zones of weakness in the lithosphere, subject to shear, compression or
extension. These angled faults solve the problem of subduction
initiation that plagues Plate Tectonics. Known as Benioff
zones, they vary in depth up to 300 km, and apparently engage the
upper mantle in its relative eastward motion, as do other deep sections
of the lithosphere. Thus the tiny measured motions of the "plates"
is the interaction of the lithosphere with the mantle
as the lithosphere drifts westward due to tidal forces.
The boundaries of
the "plates" (spreading ridges and subduction zones) formed during
the Shock Dynamics event, except for the East Pacific Rise which
formed much earlier following
Earth's grazing collision with a planetesimal that produced the
protocontinent. That "subduction zones" are as described
in presentation 10 and not sinking slabs is evident from the fact that "large,
intermediate-depth (60-300 km) earthquakes have subhorizontal rupture
planes."4
That is, earthquake faults at these depths are close to horizontal
rather than at the angle of the supposedly diving slab.
Once the sliding landmasses
slowed sufficiently, the lubricating mechanism beneath them was
lost and the continents became firmly attached to the lithosphere.
Plates
Oceanic lithosphere is roughly 100 km thick. Plate Tectonics theory
is based on the notion that the lithosphere is formed at mid-ocean
ridges and dives into the earth at trenches. This is not the
case in the Shock Dynamics theory, which says that the divisions
in the lithosphere are features that formed at the surface and extend
deep into the lithosphere, enhanced by "slow motion" differential
rotation (see below).
There
are substantial areas on the Earth that are not part of the 14 large
"plates" of Plate Tectonics theory that one hears so much
about. A study has found 38 additional "small plates"
plus a number of zones designated as "orogens" with fuzzy
plate boundaries. The largest "orogen"
stretches from Korea to the Alps. Another covers Alaska and
its connection with Canada. Most of the small plates (or microplates)
are in the region where the Australia-Southeast Asia block shattered
against Asia and scattered to the east. These exceptions to
the rule require special interpretation in Plate Tectonics theory,
and bring to mind the attempts to salvage the Earth-centered view
of the solar system by adding "epicycles" to the orbits
of planets in the days before Galileo. Bird,
Peter. 14 March 2003. An updated digital model of plate boundaries.
Geochemistry Geophysics Geosystems (G3), Vol. 4, No. 3, 52 pages.
"In the classical view, the motion of a plate was driven
from within by body forces". "However, a common
hypothesis is that microplates are externally driven, i.e., that
larger neighbor plates determine their motion." A study
of the Baja California microplate indicates that it is loosely coupled
to North America, and that 90% of its motion is from being dragged
by the Pacific plate. Plattner, C., R. Malservisi, R.
Govers. April 2009. On the plate boundary forces that drive and
resist Baja California motion. Geology, Vol. 37, No. 4, pp. 359-362.
In the Shock Dynamics
view, this kind of jostling is what is occurring today all over
the globe on every "plate" in slow motion. "Large
plates move coherently, being decoupled at the Low Velocity Zone
(LVZ). They show that the force acting on them is uniformly
distributed and not concentrated on their margins."5
In
fact, the principal driving force of Plate Tectonics theory, slab
pull on a plate margin, "would be stronger than the strength
plates can sustain under extension (pull)."5
****************
1. Anderson, Don L. 2002. Plate Tectonics as a Far-
From- Equilibrium Self-Organized System. in Plate Boundary Zones,
Geodynamics Series 30, American Geophysical Union, pp. 411-425.
2. Demouchy, Sylvie, Andrea Tommasi, Tiziana Boffa
Ballaran, Patrick Cordier. 2013. Low strength of Earth's uppermost
mantle inferred from tri-axial deformation experiments on dry olivine
crystals. Physics of the Earth and Planetary Interiors, Vol. 220,
pp. 37-49.
3. Doglioni, Carlo, Eugenio Carminati, Marco Cuffaro,
Davide Scrocca. 2007. Subduction kinematics and dynamic constraints.
Earth-Science Reviews, Vol. 83, pp. 125-175.
4. Kiser, E., M. Ishii,
C.H. Langmuir, P.M. Shearer, H. Hirose. 2011. Insights into the
mechanism of intermediate-depth earthquakes from source properties
as imaged by back projection of multiple seismic phases. Journal
of Geophysical Research, Vol. 116, B06310, pp. 1-26.
5. Riguzzi, Federica,
Giuliano Panza, Peter Varga, Carlo Doglioni. 2010. Can Earth's rotation
and tidal despinning drive plate tectonics? Tectonophysics, Vol.
484, pp. 60-73.
6. Scoppola, B., D. Boccaletti, M. Bevis, E. Carminati,
C. Doglioni. January/February 2006. The westward drift of the lithosphere:
A rotational drag? GSA Bulletin, Vol. 118, No.
1/2, pp. 199-209.
7. Smith, Alan D., Charles Lewis. 1999. Differential
rotation of lithosphere and mantle and the driving forces of plate
tectonics. Geodynamics, Vol. 28, pp. 97-116.
8. Smith, Alan D., Charles Lewis. 1999. The planet
beyond the plume hypothesis. Earth-Science Reviews, Vol. 48, pp.
135-182.
9.
Shaw, Jonathan. September-October 2016. The Plastic
Earth. Harvard Magazine, Vol. 119, No. 1, pp. 46-49. http://harvardmagazine.com/2016/09/the-plastic-earth

|